Computer Science
Mastering a prickly problem in ferrofluids
Computer simulation accurately captures the beguiling motion of a liquid magnetic material.
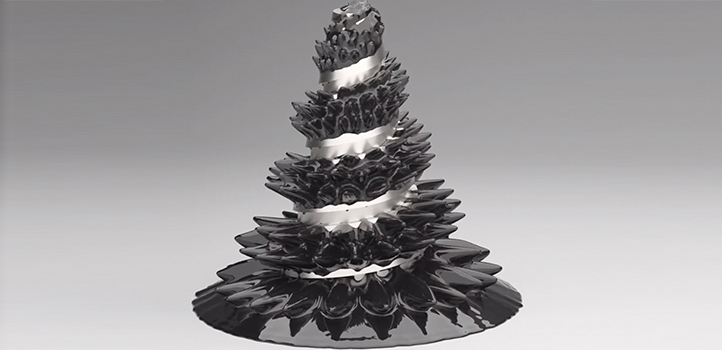
Ferrofluids, with their mesmeric display of shape-shifting spikes, are a favorite exhibit in science shows. These eye-catching examples of magnetic fields in action could become even more dramatic through computational work that captures their motion.
A KAUST research team has now developed a computer model of ferrofluid motion that could be used to design even grander ferrofluid displays. The work is a steppingstone to using simulation to inform the use of ferrofluids in a broad range of practical applications, such as medicine, acoustics, radar-absorbing materials and nanoelectronics.
KAUST researchers Dominik Michels and Libo Huang have performed computer simulations to accurately capture the behavior of ferrofluids. To see the full simulation video produced by the group visit https://www.youtube.com/watch?v=GSaipfkGpVs.
© 2019 KAUST
Ferrofluids were developed by NASA in the 1960s as a way to pump fuels in low gravity. They comprise nanoscale magnetic particles of iron-laden compounds suspended in a liquid. In the absence of a magnetic field, ferrofluids possess a perfectly smooth surface. But when a magnet is brought close to the ferrofluid, the particles rapidly align with the magnetic field, forming the characteristic spiky appearance. If a magnetic object is placed in the ferrofluid, the spikes will even climb the object before cascading back down.
Because ferrofluid behavior can be counterintuitive, simulation is the ideal way to understand their complex motion. Until now, however, the models have had several limitations, says Libo Huang, a Ph.D. student in Dominik Michels’ Computational Sciences Group within KAUST’s Visual Computing Center.
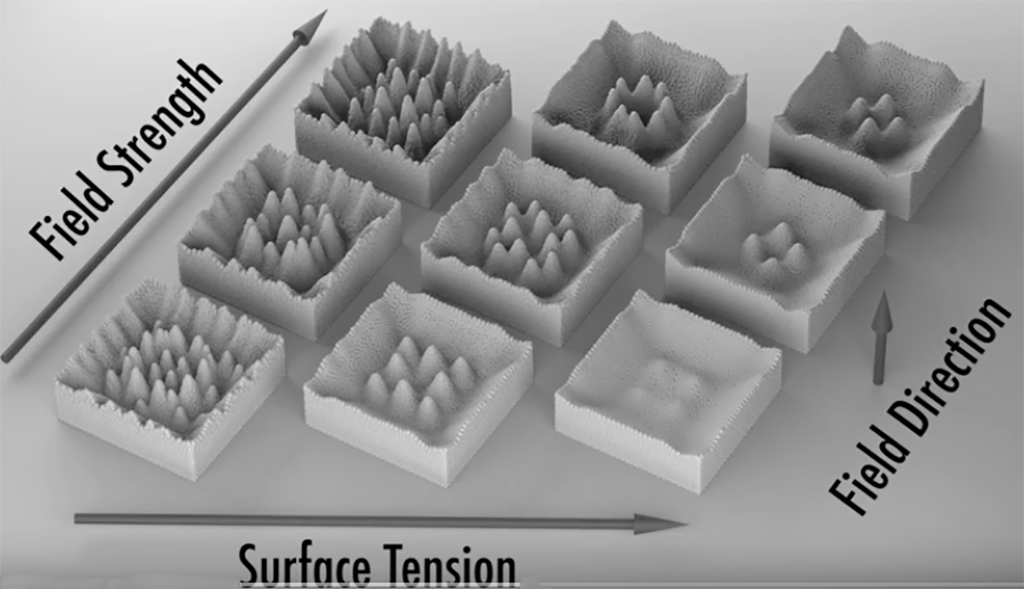
If a magnetic field is applied, the ferrofluids start to form their characteristic spikes.
© 2019 KAUST
The first challenge was to eliminate singularities in the magnetic field of existing models, Huang says. Previous models typically handled magnetic-field simulation using magnets that are infinitely small. The closer two magnets are brought together, the stronger the magnetic attraction—thus, if a magnet is infinitely small, the magnetic field strength can become infinitely large. “The center of an infinitely small magnet is called its singularity,” Huang says. Not only is the magnetic field difficult to measure at the magnet’s center, but if two singularities come close together, the forces become so large that the simulation can crash. “We derived formulas to eliminate the singularities and create much more robust numeric schemes,” Huang says.
The team also found ways to increase computational efficiency by reducing the algorithmic complexity, allowing larger simulations to be run. When the team compared their model with wet lab experiments, it reproduced the true dynamic behavior of the ferrofluid, giving a good qualitative representation that will be useful for ferrofluid sculpture design. “This opens the door for further quantitative analysis,” Huang says. Increasing the model’s accuracy further would provide new insights into fundamental ferrofluid behavior and lead to many new uses, from electronic sensors and switches to deformable mirrors for advanced telescopes.
References
- Huang, L., Haedrich, T. & Michels, D. L. On the accurate large-scale simulation of ferrofluids. ACM Transactions on Graphics 38, 4, 1 (2019).| article
You might also like
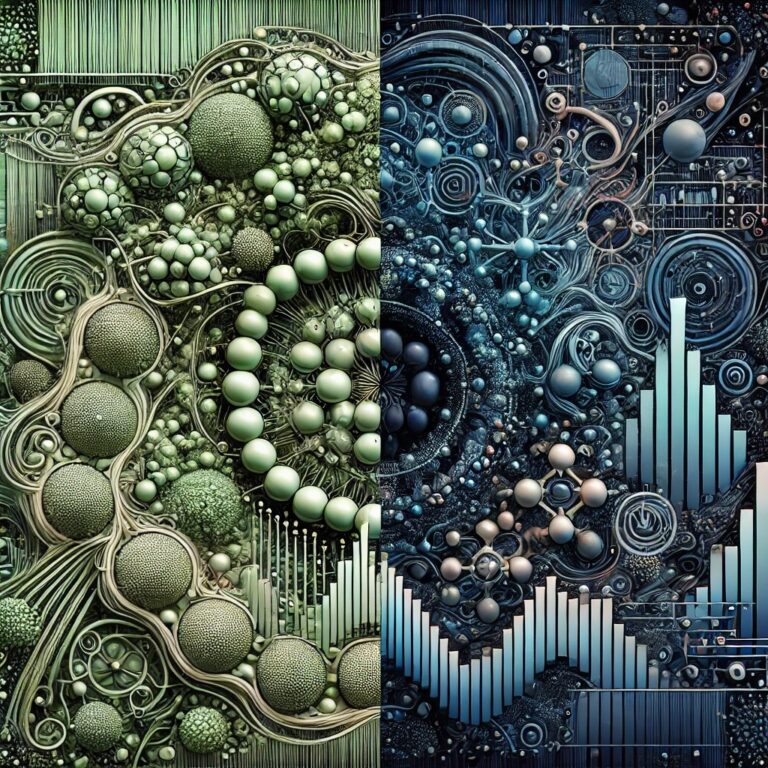
Bioscience
The theory of everything that wasn’t
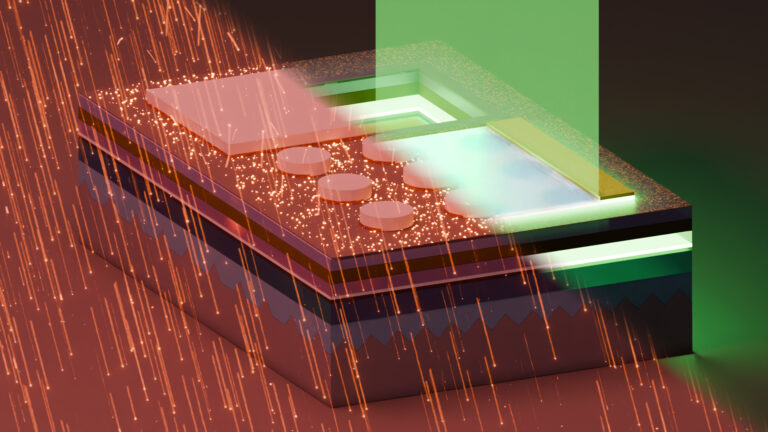
Computer Science
A new path to high-efficiency micro-LEDs
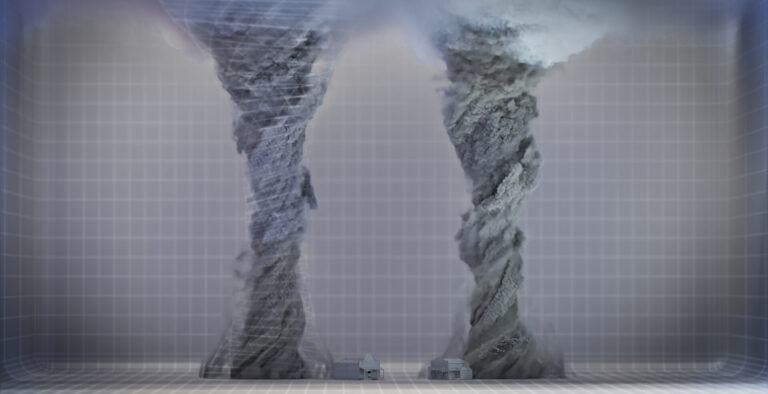
Computer Science
Cyclones meet their mathematical match
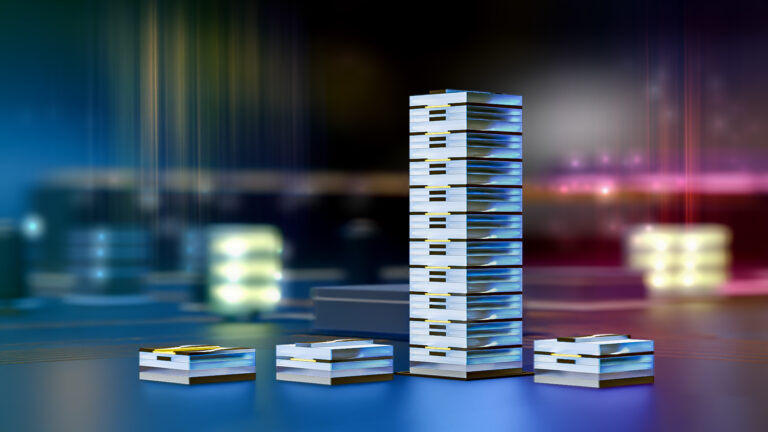
Applied Physics
Onward and upward to smaller faster devices
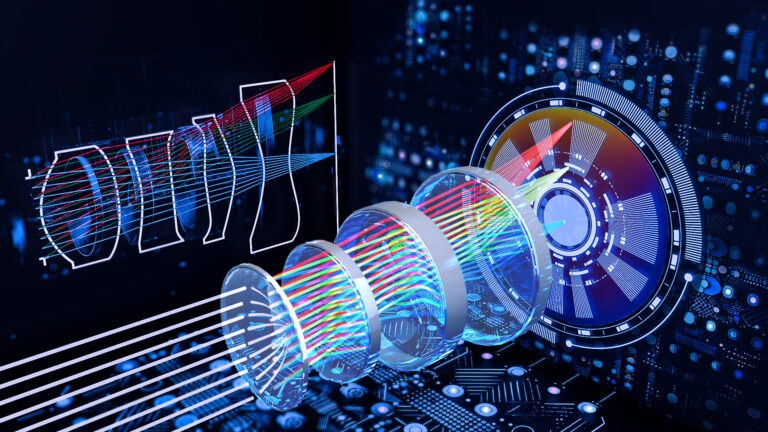
Computer Science
Deep learning improves lens design
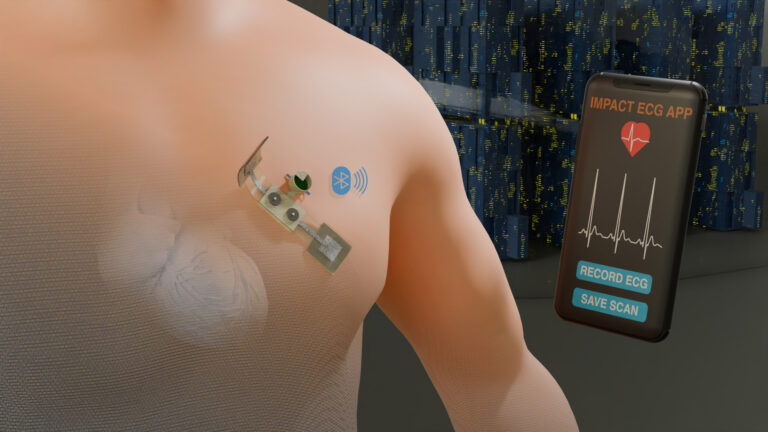
Computer Science
Better connection for monitoring helps heart patients and healthcare staff
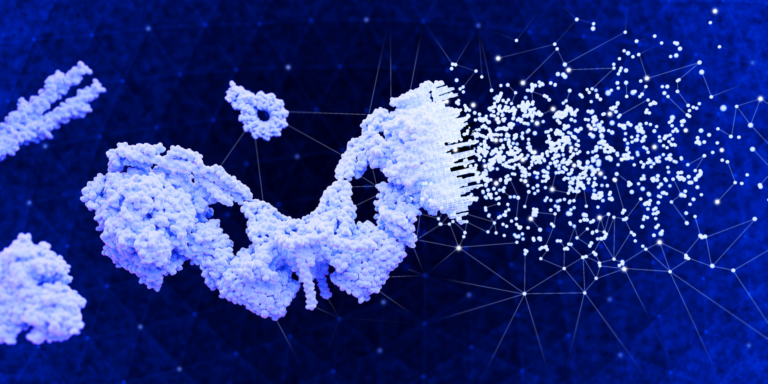
Bioengineering
AI tool predicts function of unknown proteins
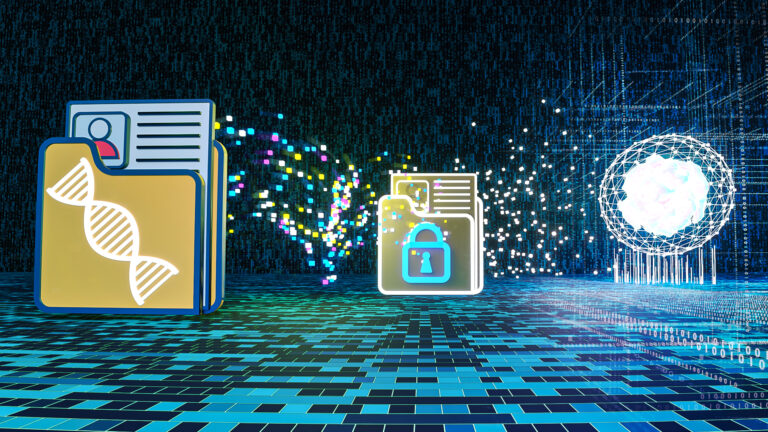
Bioengineering