Mechanical Engineering
Giant air pockets win the drag race
Creating teardrop-like gas cavities around metal spheres enables practically friction-free travel through liquids.
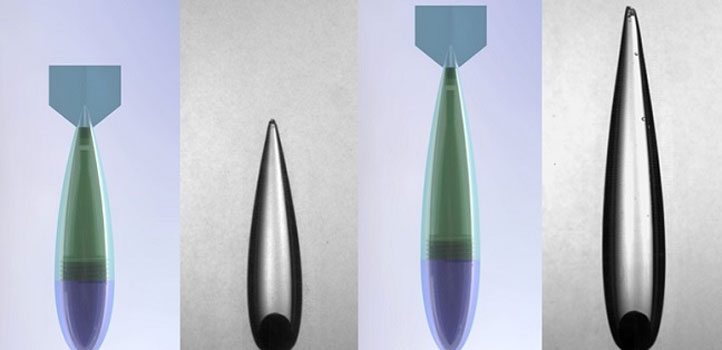
Fast-swimming fishes and deep-sea submarines share the characteristics of elongated, streamlined forms to help reduce friction and drag in water. A method developed at KAUST that spontaneously generates torpedo-shaped air pockets around objects could help oil tankers and other heavy vessels to benefit from these energy-saving tricks.
Researchers looking to minimize hydrodynamic drag often begin by modeling a sphere moving through a nonviscous, incompressible fluid. Under these ideal conditions, the drag force disappears entirely as the ball’s velocity increases—a puzzling behavior not shared by real-world objects. Understanding this paradox through controlled experiments, however, has proved difficult for nearly two centuries.
Recently, KAUST’s Ivan Vakarelski and Sigurdur Thoroddsen fired up renewed interest in drag reduction by demonstrating that metal spheres heated to 400 degrees Celsius will free-fall twice as fast in liquids as room temperature orbs. The secret lies in a thin bubble of evaporated gas that materializes around the hot balls and stops liquid molecules from sticking and creating friction. But, even after the team stabilized this gas layer with superhydrophobic coatings on the spheres, the drag forces remained much larger than was ideal.
“This prompted us to look for ways to create thicker gas layers because we suspected they would be even more effective in reducing drag,” explains Vakarelski.
Serendipity struck when the researchers noticed that the free-fall of hot spheres improved dramatically when they were dropped into the liquid instead of being released inside it. High-speed video cameras revealed that at impact, the spheres were enveloped by an air pocket that pinched off into a teardrop-like shape, 5 to 15 times the volume of the metal. The giant air pockets sped down the 2-meter-deep liquid chamber and reached a constant velocity—matching predictions of near-zero drag conditions.
“It’s intriguing how the air pocket emerges spontaneously,” says Thoroddsen. “In our earlier work, the gas layer always preserved the form of the solid bodies, but the giant gas cavity self-determines its own shape, which is very close to perfect.”
By racing their giant air cavities against identically sized plastic projectiles, the team demonstrated that gases decreased drag coefficients by an order of magnitude compared to solids. Analyses also showed the cavity’s fall velocity can be calculated exactly from the initial sphere density and the volume of trapped air.
“It’s impossible to predict how even ordinary objects like golf balls move without experiments or costly simulations,” says Vakarelski. “In contrast, we showed that a sphere-in-cavity approach can be explained by very simple equations—this could make its way into textbooks.”
References
-
Vakarelski, I. U., Klaesboer, E., Jetly, A., Mansoor, M. M., Aguirre-Pablo, A. A., Chan, D. Y. C. & Thoroddsen, S. T. Self-determined shapes and velocities of giant near-zero drag gas cavities. Science Advances 3, e1701558 (2017).| article
See the video abstract on youtube: Watch these hot balls cut through water like a knife through butter
You might also like
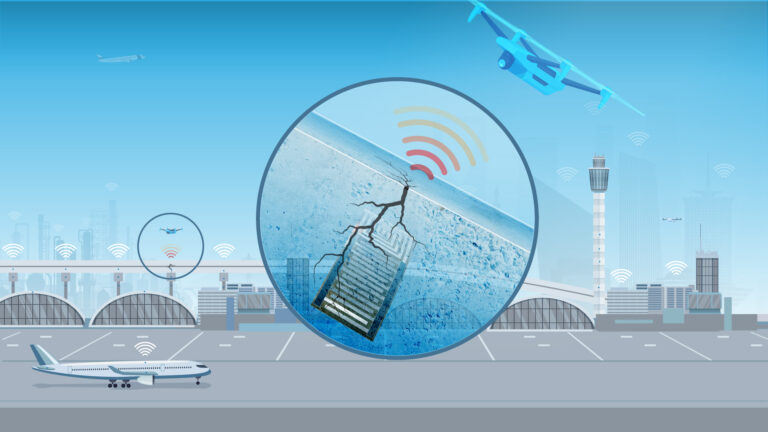
Mechanical Engineering
Innovative strain sensor design enables extreme sensitivity
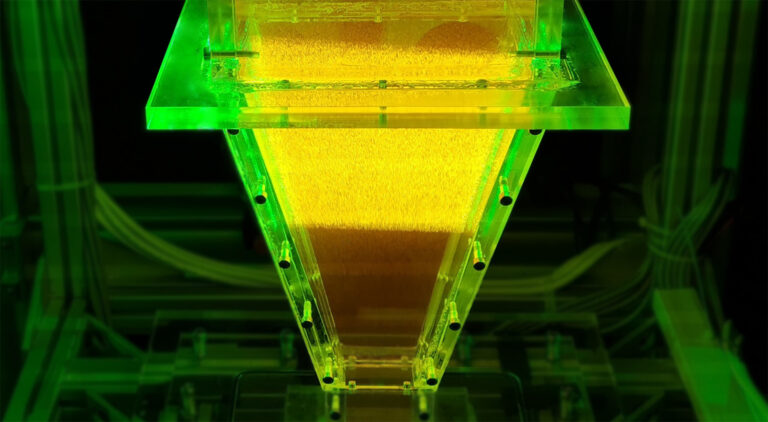
Mechanical Engineering
Turbulent flow shows surprise patterns that could help boost efficiency
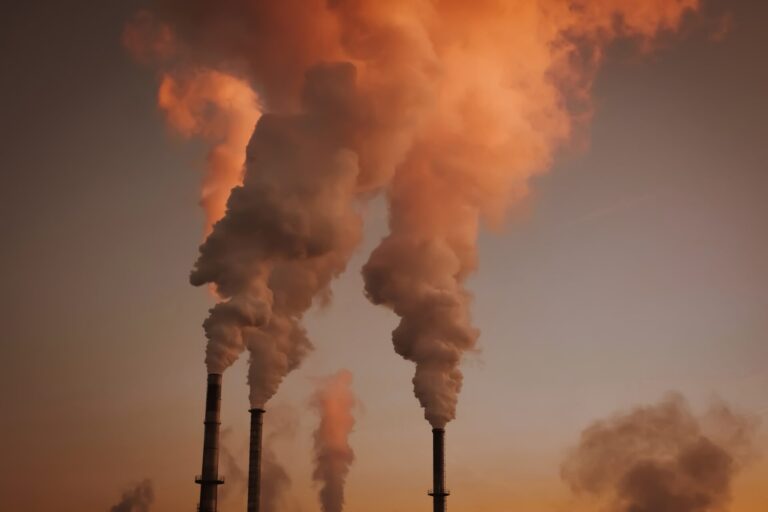
Mechanical Engineering
Machine learning model identifies gas molecules
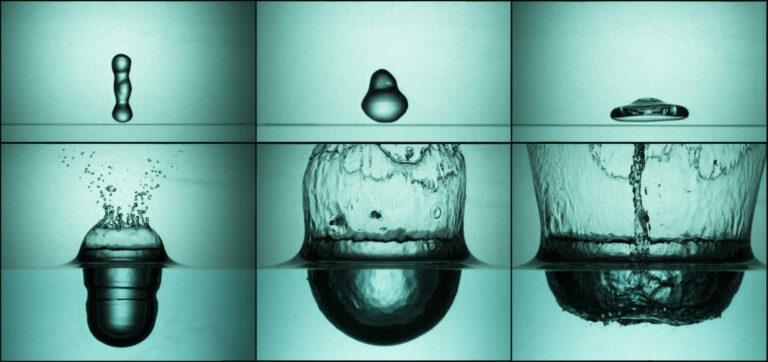
Mechanical Engineering
Making a splash: unraveling the impact of large water droplets
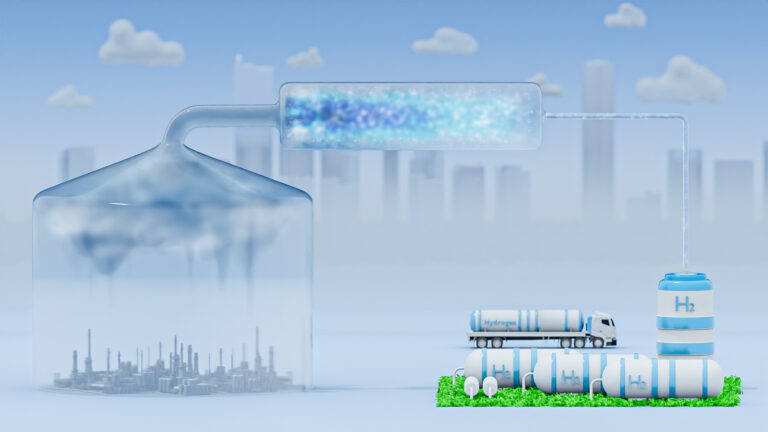
Mechanical Engineering
Sour gas has sweet potential for hydrogen production
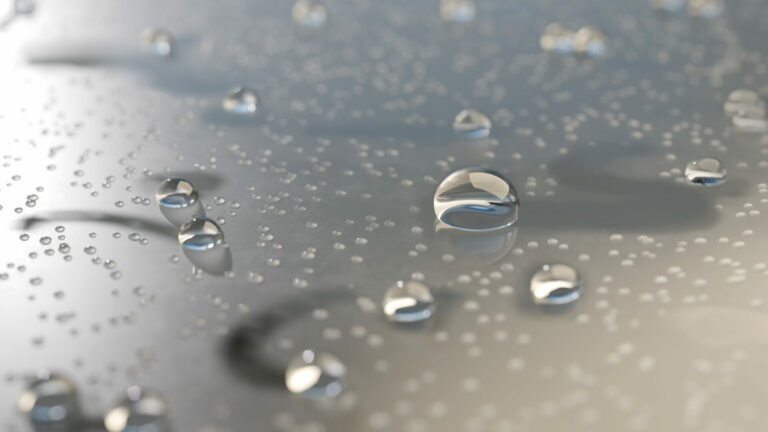
Mechanical Engineering
Dancing droplets’ new spin on water harvesting
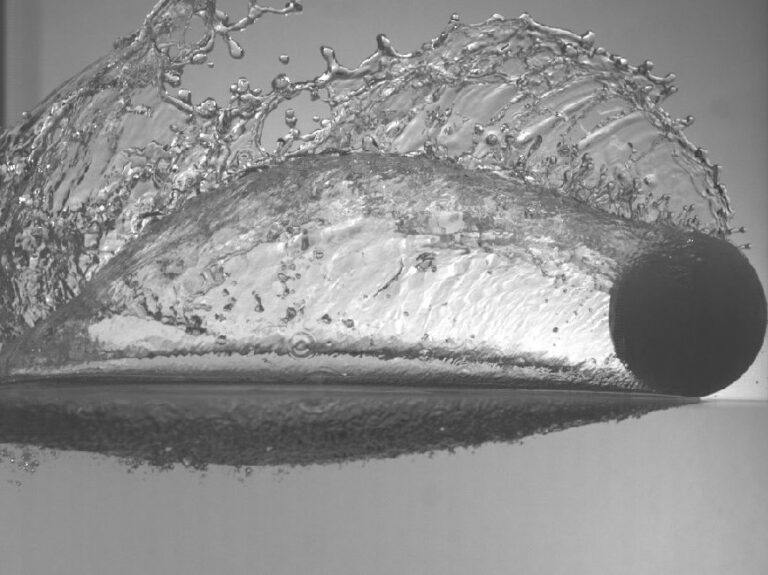
Mechanical Engineering
Underwater air pockets smooth out the bumps
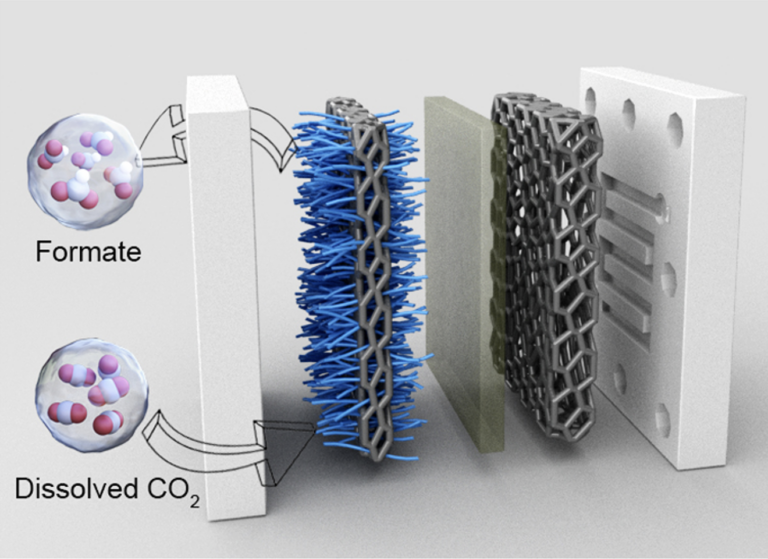
Chemistry